Rebecca A. Wingert Elizabeth and Michael Gallagher Associate Professor
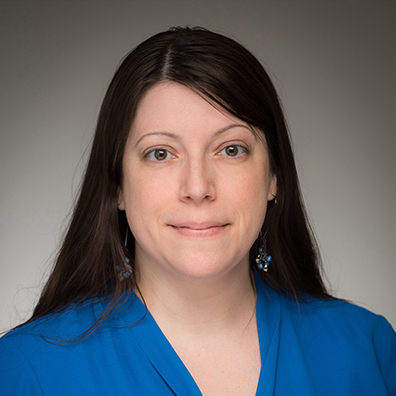
Research Interests:
The Wingert Lab studies the genetic and molecular mechanisms that control how renal stem cells accomplish kidney formation, impact kidney homeostasis, and facilitate kidney regeneration following organ injury. Understanding these processes has broad implications for identifying the basis of renal birth defects and the responses to tissue damage that lead to kidney disease. Kidney diseases are a growing global healthcare issue: they affect epidemic numbers of children and adults worldwide, and are steadily climbing in incidence. Kidney diseases can be treated with renal replacement through dialysis or organ transplant, but both strategies require life-long medical management and can involve significant complications. Knowledge obtained from studying the basic biology of the renal system can provide a valuable way to discover innovative therapies for kidney disorders.
Research in the Wingert Lab centers around the following major areas:
- Kidney Development Research: How do renal stem cells arise during development? How are nephrons constructed from renal stem/progenitor precursors? We are seeking to identify the genetic requirements for making renal cells. We utilize classical forward genetic screening and chemical genetics to study the molecules that affect in nephron formation. One benefit of these integrated approaches is that we can discover essential genes and signaling pathways that have never been implicated in renal progenitor biology. In addition, we perform expression studies to identify factors expressed by kidney cells during nephrogenesis, then assign their functional roles using reverse genetics, including knockdown and genome targeting strategies, as well as overexpression techniques to examine gain-of-function.
- Kidney Regeneration and Aging Research: How can damaged nephron components be replaced? Are there renal stem cells that can facilitate treatment of renal diseases? Can differentiated nephron cells be induced to regenerate damaged nephrons? Can the activation of kidney developmental pathways facilitate regeneration? How does the kidney age, and is the aging of renal stem cells a contributing factor to the incidence of kidney disease? We are using models of nephron injury in the embryo and adult zebrafish to discover the cell and molecular events that are required for nephron regeneration during the lifetime of the animal. Along with our nephron development studies, it is our hope that these lines of inquiry will shed novel insights into the activities of kidney cells and guide the creation of new therapeutics.
- Stem Cells, Renal Birth Defects and Rare Diseases: How do genetic alterations in renal stem cells, and their offspring, lead to conditions ranging from prevalent urogenital birth defects to rare & neglected diseases? If we uncover the pathology of these conditions, can we use this knowledge as a springboard to discover therapeutics? We are using cutting-edge genome editing strategies and other genetic tools to create novel human disease models. Of particular current interest Non-Ketotic Hyperglycinemia (NKH), a rare disease caused by inborn defects in the glycine cleavage system (GCS). NKH has an autosomal recessive inheritance pattern and is characterized by elevated glycine levels in plasma and cerebrospinal fluid (OMIM no. 605899). The GCS is an enzyme complex that consists of four proteins that catabolize the amino acid glycine, and are highly expressed in the nervous and renal systems. Mutations in the glycine decarboxylase (GLDC) gene account for most NKH cases. We are working to understand how these genetic mutations and glycine elevation affect organogenesis.
While kidney diseases are diverse in origin, many share a common aspect: alterations to the basic unit of the kidney called the nephron. Each nephron is an epithelial tube that is highly specialized to excrete metabolic waste. Nephrons contain a blood filter that collects fluid from the circulation and a long tubule that consists of a series of segments with different epithelial cell types. Nephron segments perform discrete tasks in modifying the filtrate by reabsorbing and secreting solutes—jobs that enable the retention of desirable nutrients and export of metabolic wastes. Human kidneys are architecturally complex because they contain many nephrons (ranging from several hundred thousand to over one million) that have intricate loops and convolutions, and are organized in arbor-like arrays. The signaling pathways that control how renal stem cells give rise to nephrons during kidney development are poorly understood. Part of the reason for this is that the architecture and internal location of the kidney pose challenges for studying nephron development and dysfunction in mammals. For some time, there has been experimental evidence that the kidney exhibits a limited capacity to regenerate. Damage to epithelial cells in nephrons can be followed by a local regenerative response in which new nephron epithelial cells are made. However, the molecular mechanisms that enable this type of nephron regeneration remain largely enigmatic, and their discovery is hampered by the aforementioned limitations of existing mammalian models.
To study how nephrons are made during development, regeneration, and in disease models, we use the zebrafish, Danio rerio. The zebrafish is an outstanding model for kidney research for numerous reasons. First, zebrafish are a vertebrate species and share many similarities with more complex vertebrates like mammals. For example, there is a high degree of conservation between gene function and basic cellular processes between zebrafish and mammals. Zebrafish embryos develop outside the mother and are optically transparent, enabling the direct visualization of organ development. The embryo forms an anatomically simple kidney that is made up of two nephrons, while the zebrafish adult kidney displays complex nephron arrangements analogous to other higher vertebrates. Zebrafish nephrons are comprised of segments with epithelial cells that share gene expression signatures and ultrastructural traits with the segments that typify vertebrate nephrons. Importantly, there is a diverse arsenal of molecular tools now available to the zebrafish researcher, and these enable high-resolution study of cell biology and genetic analysis.
Biography:
- Chair, Institutional Animal Care and Use Committee, 2017-Present
- Director of Graduate Studies, Department of Biological Sciences, Notre Dame 2016–Present
- Associate Professor, Department of Biological Sciences, Notre Dame 2016–Present
- Association for Women in Science (AWIS), Notre Dame Chapter, Faculty Advisor 2013–Present
- Gallagher Family Professorship in Stem Cell Biology 2012–Present
- Assistant Professor, Department of Biological Sciences, Notre Dame 2010–2016
- Harvard Stem Cell Institute, Affiliated Faculty 2008–2010
- Board of Tutors in Biochemical Sciences, Harvard University 2008–2010
- Instructor in Medicine, Harvard Medical School 2007–2010
- Continuing Education/Special Programs Instructor, Harvard University 2003–2007
- Postdoctoral Fellow, Harvard Medical School and Massachusetts General Hospital 2005–2010
- Ph.D. Cell and Developmental Biology, Harvard University 1999–2005
- B.A. English, B.S. Biology, Muhlenberg College 1995–1999
Recent Papers:
- Wesselman HM, Flores-Mireles A, Wingert RA. 2021. Esrrγa regulates nephron development and ciliogenesis by controlling prostaglandin synthesis and cooperation with Ppargc1a. https://www.biorxiv.org/content/10.1101/2021.11.17.468871v1
- Drummond BE, Chambers BE, Wesselman HM, Ulrich M, , Gerlach GF , Kroeger PT , Leshchiner I, Goessling W, Wingert RA. 2021. osr1 maintains renal progenitors and regulates podocyte development by promoting wnt2ba through antagonism of hand2. https://www.biorxiv.org/content/10.1101/2020.12.21.423845v1
- Chambers JM, Addiego A, Flores-Mireles AL, Wingert RA. 2020. Ppargc1a controls ciliated cell development by regulating prostaglandin biosynthesis. Cell Reports 33(6): 108370. DOI: 10.1016/j.celrep.2020.108370
- Chambers BE, Clark EG, Gatz AE, Wingert RA. 2020. Kctd15 regulates nephron segment development by repressing Tfap2a activity. Development 147(23): dev191973. DOI: 10.1242/dev.191973
- Chambers JM, Wingert RA. 2020. PGC-1a in disease: recent renal insights into a versatile metabolic regulator. Cells 9(10):2234. DOI: 10.3390/cells9102234
- Chambers JM, Wingert RA. 2020. Advances in understanding vertebrate nephrogenesis. Tissue Barriers 8(4):1832844. DOI: 10.1080/21688370.2020.1832844
- Chambers JM, Wingert RA. 2020. New zebrafish model for monitoring proximal tubule physiology in genetic and acquired renal Fanconi syndromes. Kidney International 97(6):1097-1099. DOI: 10.1016/j.kint.2020.02.014
- Marra AN, Chambers BE, Chambers JM, Drummond BE, Adeeb BD, Wesselman HM, Morales EE, Handa N, Pettini T, Ronshaugen M, Wingert RA. 2019. Visualizing gene expression during zebrafish pronephros development and regeneration. Methods in Cell Biology 154: 183-215. DOI: 10.1016/bs.mcb.2019.06.003
- Chambers BE, Gerlach GF, Clark EG, Chen K, Levesque A, Leshchiner I, Goessling W, Wingert RA. 2019. Tfap2a is a novel gatekeeper of nephron differentiation during kidney development. Development 146(13): dev172387. DOI: 10.1242/dev.172387
- Chambers BE , Wingert RA. 2019. A zebrafish tale of parabiosis, podocytes and proteinuria. Kidney International 96(2): 272-275. DOI: 10.1016/j.kint.2019.04.041
- Marra AN, Cheng CN, Adeeb BA, Addiego A, Wesselman HM, Chambers BE, Chambers JM, Wingert RA. 2019. Iroquois transcription factor irx2a is required for multiciliated and transporter cell fate decisions during zebrafish pronephros development. Scientific Reports 9(1):6454. DOI: 10.1038/s41598-019-42943-y
- Marra AN, Adeeb BD, Chambers BE, Drummond BE, Ulrich M, Addiego A, Springer M, Poureetezadi SJ, Chambers JM, Ronshaugen M, Wingert RA. 2019. Prostaglandin signaling regulates renal multiciliated cell specification and maturation. Proceedings of the National Academy of Sciences of the United States of America 116(17): 8409-8418. DOI: 10.1073/pnas.1813492116
- Chambers BE g, Wingert RA. 2019. Mechanisms of nephrogenesis revealed by zebrafish chemical screen: prostaglandin signaling modulates nephron progenitor fate. Nephron 143(1): 68-76. DOI: 10.1159/000501037
- Chambers BE, Wingert RA. 2019. A zebrafish tale of parabiosis, podocytes and proteinuria. Kidney International 96(2): 272-275. DOI: 10.1016/j.kint.2019.04.041
- Chambers BE, Wingert RA. 2019. Nephron repair: powered by anaerobic energy metabolism. Annals of Translational Medicine 7(Suppl 1): S28. DOI: 10.21037/atm.2019.01.73.
- Morales EE, Handa N, Drummond BE, Chambers JM, Marra AN, Addiego A, Wingert RA. 2018. Homeogene emx1 is required for nephron distal segment development in zebrafish. Scientific Reports 8(1): 18038. DOI: 10.1038/s41598-018-36061-4
- Chambers J, Poureetezadi SJ, Addiego A, Lahne M, Wingert RA. 2018. ppargc1a controls nephron segmentation during zebrafish embryonic kidney ontogeny. Elife 7: e40266. DOI: 10.7554/eLife.40266